The Arctic is warming at a rate of four times the rest of the globe. The increasing rates of Arctic coastal erosion, driven by climate change, have endangered essential infrastructure and indigenous communities. Despite the Arctic accounting for a third of the world’s coastline, existing tools cannot accurately explain unpredictable, storm-induced incidents. Funded by Laboratory Directed Research and Development (LDRD) and Biological and Environmental Research (BER) programs, and in collaboration with University of Texas at Austin, University of Alaska Fairbanks, US Geological Survey (USGS) and Integral Consulting, scientists from Sandia have established an innovative, multi-physics finite element simulation framework, known as the Arctic Coastal Erosion (ACE) model, for numerically modeling Arctic coastal permafrost. The ACE model enhances comprehension of coastal erosion processes, contributes to predictions of erosion-driven biogeochemical and sediment burdens, and can be used to help assess infrastructure vulnerability.
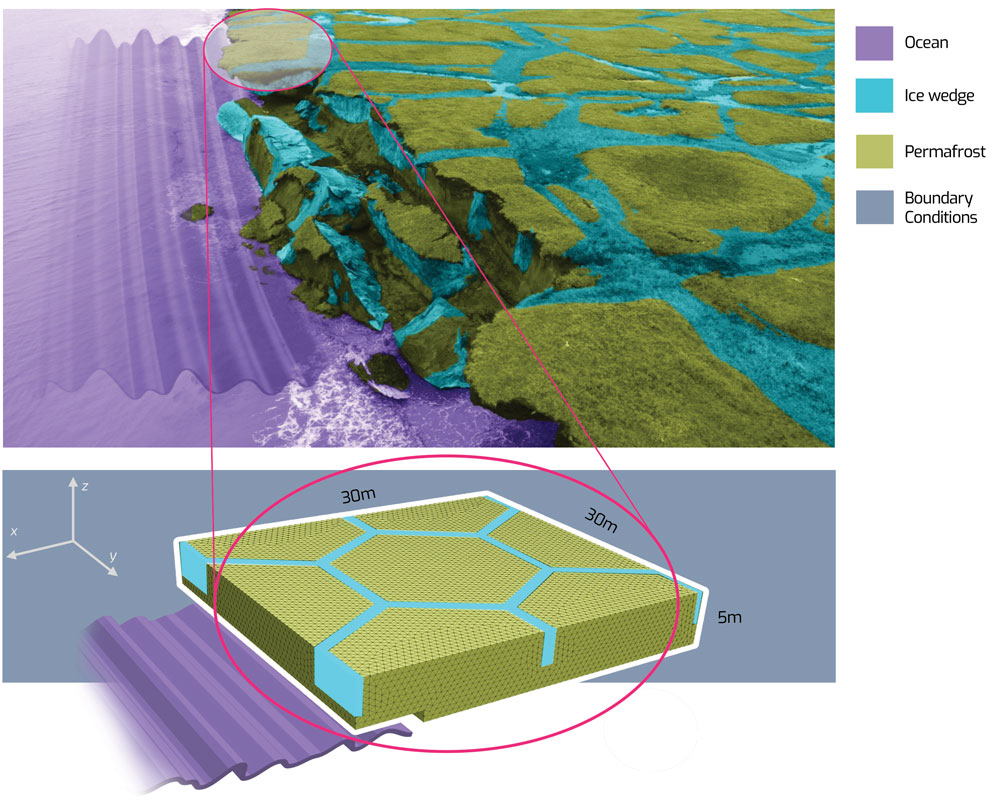
Existing state-of-the-art permafrost models
When the Energy and Homeland Security LDRD project that led to the creation of ACE began in 2017, no existing tools could accurately predict Arctic coastal erosion. Many state-of-the-art permafrost models were based on trend projections and/or empirical relationships, lacking consideration for the governing partial differential equations, or PDEs. While a few limited PDE-based models were available, these were predominantly thermal models that ignored the crucial role of mechanics and deformation in the permafrost degradation process. Most of these models assumed a specific erosion type (such as block failure) and failed to account for permafrost geophysics or geomorphologies. Further, these models tended to not realistically account for the changing conditions driving the erosion or the atmospheric and oceanographic changes; they simply relied on trend projections or aggregate environmental changes incapable of resolving the storm-driven episodic events by which significant amounts of erosion are forced. In contrast to earlier models, ACE is a systems model that ingests accurately modeled boundary conditions at relevant spatio-temporal scales into a 3D PDE-based model that incorporates interlinked thermo-mechanical effects on a discretized permafrost geomorphology, consisting of an ice-wedge polygon morphology (see Figure 1).
A coupled thermo-mechanical finite element formulation with element erosion/failure
The ACE model’s core terrestrial modeling component comprises two main elements: (1) a solid mechanics model, which calculates the 3D stress, strain and displacement fields of the evolving permafrost based on a frozen water content-dependent plasticity model, and (2) an innovative thermal model that regulates the heat conduction and phase transformation within the permafrost. The coupling of these two physics sets occurs through a sequential thermo-mechanical coupling scheme, developed within the Albany-Laboratory for Computational Mechanics, or LCM, open-source finite element code (https://github.com/sandialabs/LCM).
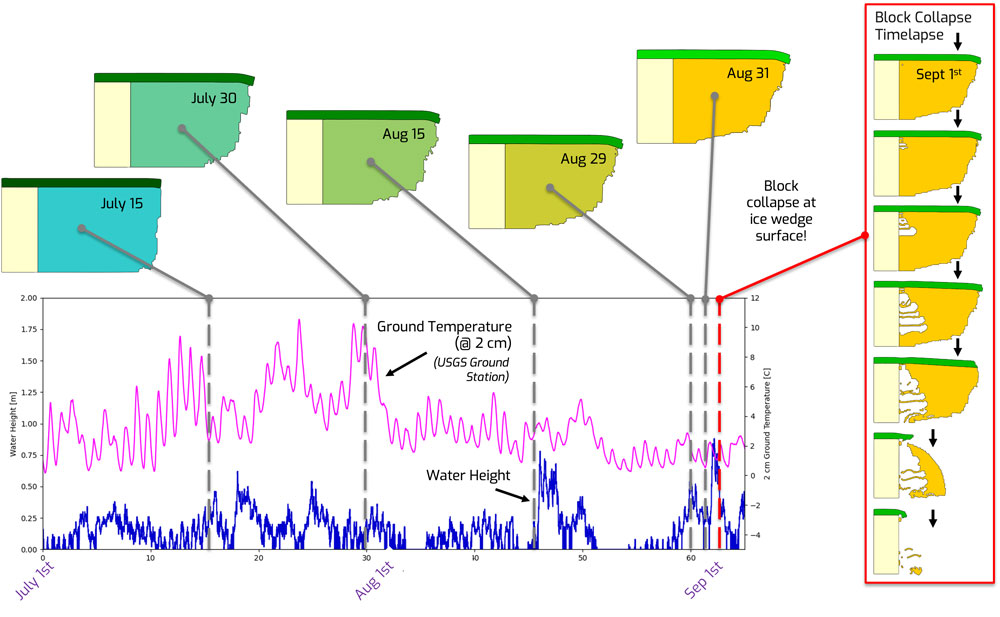
The mechanical component of ACE includes plasticity, modeling the mechanical strength of a permafrost bluff as a function of its thermal state through calculations by the thermal component of ACE. More specifically, the strain and displacement fields of the underlying permafrost are coupled to a novel thermal model governing the 3D heat conduction and solid-liquid phase change occurring within the permafrost.
The terrestrial state is forced using realistic atmospheric and oceanic boundary conditions. An oceanographic modeling suite (consisting of WAVEWATCH III, Delft3D-FLOW, and Delft3D-WAVE) produced time-dependent surge and run-up boundary conditions for the terrestrial model. This suite utilizes historical and projected atmospheric conditions from Earth System Models, or ESMs, to boundary conditions; these ESMs also provide the atmospheric conditions to the terrestrial model. The material and thermal parameter for frozen soil within ACE are informed by experiments on natural cores collected from Drew Point, Alaska, conducted by Sandia’s Geomechanics Laboratory. Compressive and tensile experiments were performed at near thaw conditions to define failure and elastic moduli-based ice saturation levels.
Unique capabilities of the ACE model
The ACE model offers several unique capabilities. First, it can simulate permafrost erosion events by dynamically removing elements from the underlying finite element mesh based on physically motivated failure criteria. Moreover, because the ACE thermo-mechanical model captures permafrost deformation according to ice saturation-dependent stresses, it naturally captures permafrost subsidence as heat flow to and from permafrost. The ACE model hence differs significantly from previous approaches, as it enables failure from any permissible deformation (block failure, thermo-denudation, thermo-abrasion), and defines failure modes based on constitutive relationships innate in the underlying physics, instead of predetermined failure planes, boundary condition approximations, critical niche depth or empirical relationships.
Utilization of Sandia HPC
The ACE thermo-mechanical model is implemented within the Albany-LCM HPC finite element code, which is developed and maintained on a variety of Sandia HPC machines, including Common Engineering Environment (CEE) and Skybridge. Nightly testing is performed on all targeted architectures to ensure code quality and performance are maintained. The ACE team is currently in the process of calibrating and validating their model using observational data collected in northern Alaska during a 2018-2019 field campaign and Sandia HPC (Figure 2). The projected oceanographic boundary conditions through 2050 were also generated on Sandia HPC.
Future extensions and applications
Once the ACE thermo-mechanical model is calibrated and validated, the ACE team intends to expand the model toward addressing national security issues linked with climate change and permafrost decline, such as threats to crucial Arctic infrastructure. As part of the BER InteRFACE project (https://arcticinterface.org/), techniques to upscale model outputs are being pursued toward studying permafrost demise along the entire Alaskan coastline, among other locations.
Because the ACE thermo-mechanical model is able to naturally capture permafrost, together with its implementation within the Albany-LCM finite element HPC code, the model is particularly well-suited to serve as the foundation for a groundbreaking framework that enables the simulation of critical infrastructure placed on top of permafrost. The team is currently looking for funding opportunities to create a unique simulation tool that would couple the ACE permafrost model to a mechanics-based model of critical infrastructure placed on top of permafrost. The new model would enable assessments of climate change-induced risk to a variety of infrastructure-permafrost combinations, toward predicting likely failure mechanisms and proposing possible solutions to decrease risk. The team is additionally interested in developing, through a series of laboratory experiments involving synthetic soil samples, a universal constitutive model for frozen soils that would be broadly applicable across the Artic.
References [1] J. Frederick, A. Mota, I. Tezaur, D. Bull. “A thermo-mechanical terrestrial model of Arctic coastal erosion”, J. Comput. Appl. Math. 397 113533, 2021.
[2] M. Thomas, A. Mota, B. Jones, C. Choens, J. Frederick, D. Bull. Geometric and material variability influences stress states relevant to coastal permafrost bluff failure. Frontiers in Earth Science, 8:143, 2020.
[3] D. Bull, C. Flanary, C. Jones, J. Frederick, A. Mota, I. Tezaur, J. Kasper, E. Brown, B. Jones, M. Jones, E. Bristol, C. Choens, C. Connoly, J. McClelland. “Arctic Coastal Erosion: Modeling and Experimentation”. Sand No. 2020-10223. Sandia National Laboratories, Albuquerque, NM, 2020.