Scientists detect gases from fractured rock
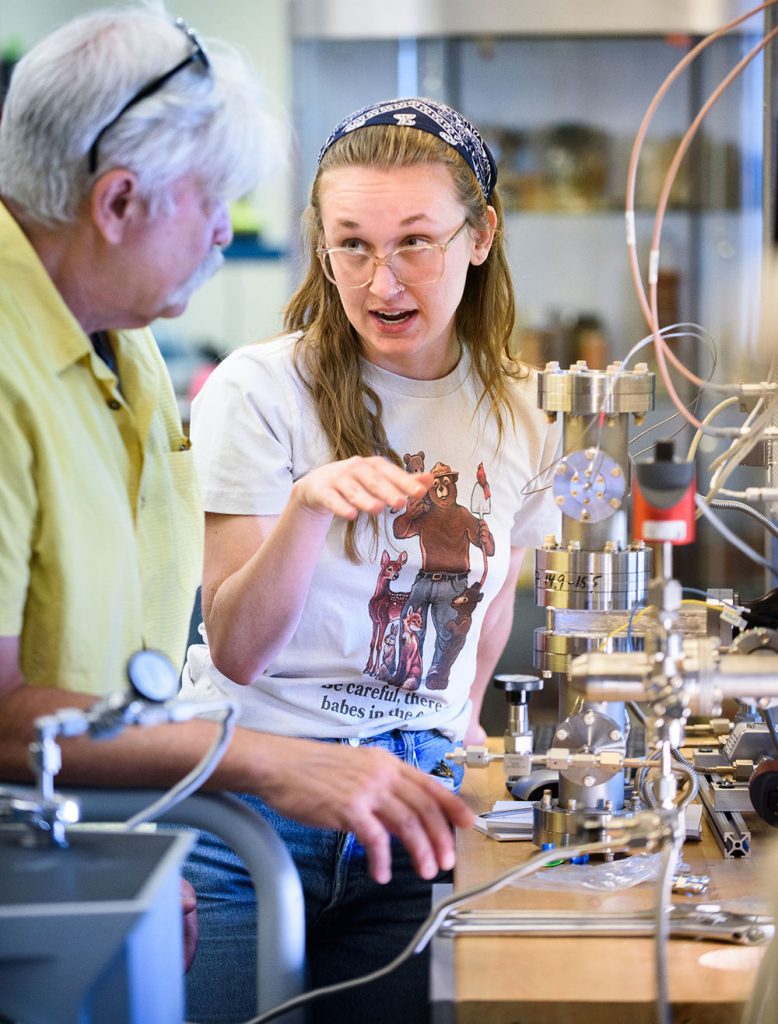
Geoscientists have detected specific gases being released from fractured rocks in real time after a series of small chemical explosions set underground.
This fundamental research, led by Sandia geoscientist Steve Bauer, could one day improve the prediction of earthquakes or detection of underground explosions.
“In the different shots, we were able to measure different relative amounts of noble gases, which we hope is consistent with the amount of deformation caused by the explosion,” said Steve, who recently retired. “The chemical explosions had the same amount of TNT equivalent, but produced different amounts of liquids and gases, so they deformed the rock to different extents. My pie-in-the-sky goal is to measure the amount of noble gas at a location after an explosion or earthquake and then be able to understand the amount of strain the rock has experienced.”
Fundamentals of noble gases and lab tests
Inert noble gases, including helium, neon and argon, naturally form underground as radioactive elements in rock decay into lighter, more stable elements. These gases become trapped in the structure of nearby rock and some of it can be released when the rock is cracked, whether by a natural earthquake or a human-caused underground explosion, Steve said.
“The release of noble gases had been observed in the field in relation to earthquake activity, so we thought we would have a good chance of making these measurements in the field,” Steve said.
But before Steve and his colleagues Scott Broome, a Sandia geoscientist, and Payton Gardner, a geosciences professor at University of Montana, conducted their experiments in the field, they deformed, or crushed, a two-inch-diameter cylinder of rock along three axes — the top-bottom, left-right and front-back — inside a sealed container.
“In the lab we learned that when we stress or strain different types of rock to a certain point, it begins to fracture, and at the time the rock fractures, it releases noble gases that we can sense with mass spectrometry,” Steve said. “We can sense the gas coming out when the rock is fractured internally. We’ve done this experiment with numerous types of rock: tuff, salt, granite, other igneous and sedimentary rocks. We were probably the first to make the gas measurements in real time as the rock was being deformed.”
Mass spectrometry is a powerful method for measuring ions to either determine what an unknown material is made up of, or as in this case, to precisely determine the ratios of different elements in a sample. The team used a quadrupole mass spectrometer for these tests.
Then they moved on to in-field experiments, using the same powerful method to detect noble gases.
Moving to field tests
The team was involved in a collaboration where three underground explosions were conducted at the Energetic Materials Research and Testing Center outside of Socorro, New Mexico, in the Blue Canyon Dome made of rhyolite rock.
Each of the test explosions used a different explosive compound but the same basic setup. The explosion was set off down a well less than a foot across at a depth of 130-200 feet. Arrayed 15-23 feet away around this central hole were eight other monitoring wells that went down about 200 feet.
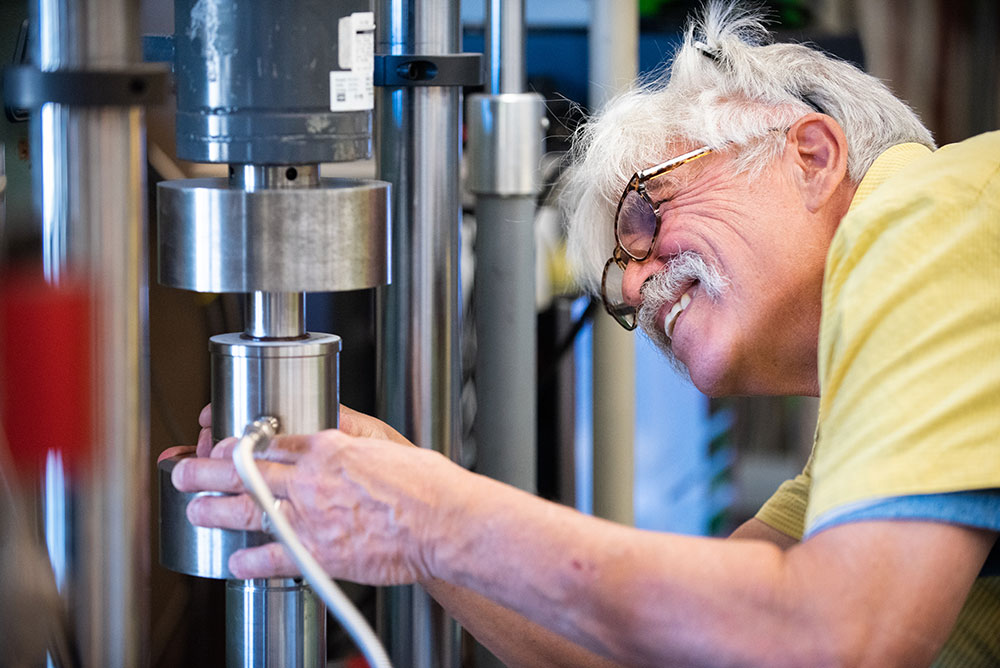
Steve’s team monitored the naturally formed noble gases and trace gases after the chemical explosions in these wells while other teams from Sandia and Pacific Northwest National Laboratory looked at large-scale changes in the permeability of the rock, the release of radon, as well as traditional and emerging seismological measurements.
“Conducting these experiments in the field is technically challenging,” Scott said. “To measure these gases, you need equipment that is very sensitive to pressure fluctuations and inherently, when you explode rock underground, it creates large pressure fluctuations. You need to protect the mass spectrometer from the initial shock of the experiment. We have seen noble gas released from these tests, and we have also not seen it. We’ve been able to publish on both of these observations. This is pretty fundamental research.”
The first explosion didn’t release any noble gases that could be recorded, which led the team to infer that the explosion caused shifts in existing fractures underground, rather than creating new fractures, Scott said. They published their results and conclusions from this first test in 2021 in the scientific journal Geofluids.
“In the two additional explosions we got much better data; we were able to document the release of noble gases as a function of time after the explosions,” Steve said. “After the explosion, it takes a certain amount of time for the gases to travel through the damaged rock to get to our detection array.”
They plan to continue their work with another set of experiments in the field, in a different type of rock, called tuff. For this set, they will not measure the gases in real time, but instead collect samples from different locations and at different times after the explosions for later analysis.
In addition to monitoring earthquakes and underground explosions, improved understanding of how and why rocks fracture could also be valuable for enhanced geothermal systems and strain detection in unconventional oil and gas production.
This work was started with funding from Sandia’s Laboratory Directed Research and Development program in 2016 and continues with support from NNSA’s Office of Defense Nuclear Nonproliferation Research and Development.