Standardized screening aids NRC risk assessment in advanced reactor licensing
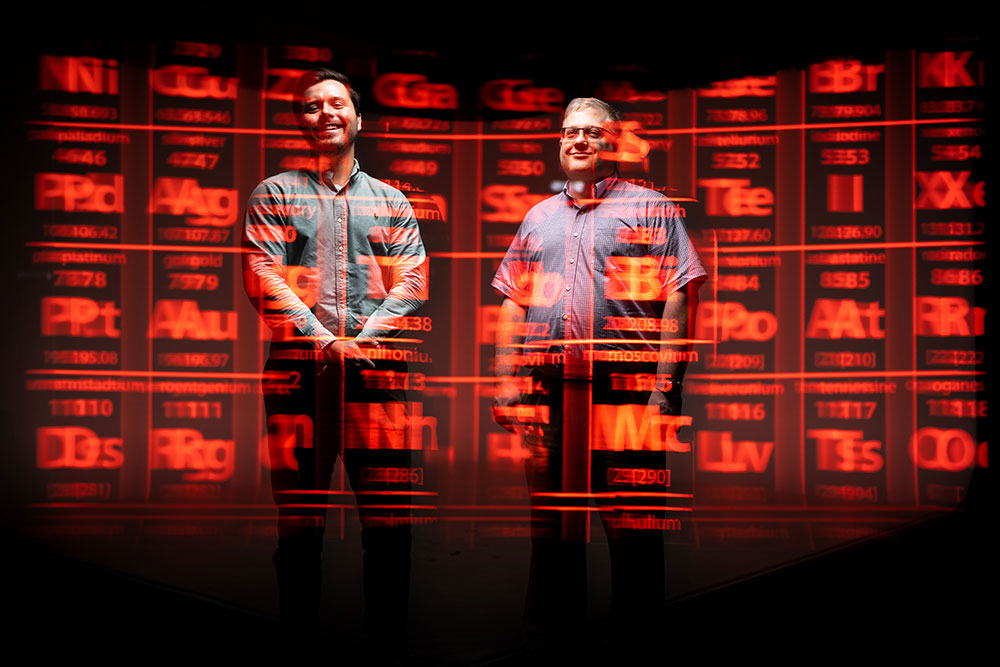
Nuclear power is a significant source of steady carbon-neutral electricity, and advanced reactors can add more of it to the U.S. grid, which is vital for the environment and economy.
For decades, Sandia has supported the Nuclear Regulatory Commission in its role of regulating and licensing nuclear reactors. With many advanced nuclear reactor designs being developed for potential licensing, this support is as important as ever, said Sandia geosciences engineer Kyle Clavier.
One of Sandia’s newest aids for NRC licensing efforts is a standardized screening method to determine the most important radioactive isotopes that could leave an advanced reactor site in the unlikely event of an accident. The Sandia team recently applied it to a conceptual design for a heat pipe reactor and shared the results with the commission and greater scientific community.
Radioactive isotopes are unstable forms of elements that release energy in various forms of potentially harmful radiation as part of the process of becoming more stable isotopes. For example, naturally occurring radon-222 is a product of the decay of uranium and in turn decays into polonium-218, releasing alpha radiation. This decay process is particularly harmful if it occurs in someone’s lungs, which is why the Environmental Protection Agency urges homeowners to test for the buildup of radon gas in their houses.
“We’re having a nuclear renaissance right now, where quite a few new reactor designs are coming out that promise to have more passive safety features, be more modular and have other advantages over conventional nuclear reactors,” said Kyle, who has worked on the method and applying it to an example reactor design. “We are working to provide the NRC the tools it needs to make sure that when these new advanced reactors are licensed, the NRC can accurately quantify the potential risks and thus ensure that the reactors are safe for operation.”
A heat pipe reactor is an advanced design that uses a substance such as an inert gas or liquid metal to cool the core. This means that the reactor could potentially need a lot less water than light-water reactors, which use normal water to cool the nuclear fuel and produce steam to generate electricity, said Dan Clayton, a Sandia nuclear engineer also heavily involved in the project. Light-water reactors are the most common type of nuclear power plant design.
Additionally, the fluid in the heat pipes does not need any moving parts, such as valves or pumps, to regulate the core’s temperature, which means they do not require electricity to ensure the safety of the reactor.
Winnowing down the list
The team’s screening method starts with an inventory of potentially thousands of radioactive isotopes produced by a nuclear reactor and sorts them to determine the radioactive isotopes that pose the most risk to humans and the environment in the unlikely case of an accident.
In the example of a heat pipe reactor, they started with Oak Ridge National Laboratory’s preliminary list of more than 1,200 radioactive isotopes predicted to be generated by this kind of reactor. First, they removed the isotopes that decay very quickly, said Kyle.
For example, rhodium-106, a form of the element rhodium with 45 protons and 61 neutrons, has a half-life of 29.9 seconds. This means that after 30 seconds, half of the initial amount of rhodium-106 will have become palladium-106 — a stable metallic element with 46 protons and 60 neutrons — releasing beta radiation. After a minute, only a quarter of the initial amount of rhodium-106 will remain. Even if an accident were to occur rapidly, very little rhodium-106 would remain to affect people or the environment.
Then the team pared down the list by removing very rare isotopes, specifically isotopes that comprised less than 0.0001% of the total radioactivity of the inventory.
“One of the reasons behind this screening method is to identify the isotopes that are actually important because we have limited resources,” Dan said. “We want to be able to focus on the new radioactive isotopes that might need to be studied further to ensure the NRC can appropriately and efficiently review safety applications. By screening out the rare isotopes, we can decrease the cost and time needed to evaluate these new reactors without compromising safety.”
These first two screening methods were selected to be similar to those used in the 1970s and 1980s to determine the radioactive isotopes of interest for light-water reactors, said Kyle. Using these two screenings, the team produced a targeted list of 110 radioactive isotopes for further study.
Quantifying health impacts
The team then took a set amount of each of the radioactive isotopes and determined the resulting radiation doses using values from an EPA report. The dose is a numerical representation of the health impacts of exposure from that radioactive isotope, Kyle said. Specifically, they were able to use advanced computer codes like Maccs to calculate the transport of radioactive isotopes through the environment and the hazard posed by the isotopes of interest on the bone marrow and the lungs — two organs that provide a good picture of the overall health impacts of radiation exposure.
Combining these dose values from a set amount of radioactive isotope with the proportion of that isotope present in the heat pipe reactor inventory, they were able to calculate both short-term and long-term health impacts of the studied radioactive isotopes. Then they compared these dose values with equivalent doses of iodine-131 and cesium-137.
Iodine-131 is the radioactive isotope that poses the most short-term risk from an accident at conventional nuclear power reactors. Therefore, potassium iodide tablets — which block the body’s intake of radioactive iodine — are distributed near U.S. nuclear power plants just in case. Cesium-137 is the isotope that provides a good representation of the long-term risk from an accident at conventional nuclear power reactor, Kyle said.
They found over a dozen radioactive isotopes present in the heat pipe reactor inventory that could pose short-term health risks on par with iodine-131. Four radioactive isotopes present in the heat pipe reactor inventory could pose long-term health risks on par with cesium-137.
While this research suggests that these isotopes may be important for future consequence assessment of heat pipe reactors, more research is needed to refine the heat pipe reactor inventory and determine the proportions of isotopes that could be released during a possible accident, Kyle said.
In the future, scientists from Sandia and the NRC will be able to use this transparent and traceable method to determine targeted lists of radioactive isotopes of concern from the inventories of other advanced nuclear reactor designs, said Kyle and Dan.
“We found a number of radioactive isotopes, beyond those we already consider for light-water reactors, that are important for consequence analysis,” Kyle said. “However, the purpose of the study was to develop a methodology that you could apply to any reactor design, as the information on the inventories of those reactors become available. The heat pipe reactor was chosen to demonstrate the method. It’s exciting to be able to use our expertise to assist U.S. regulatory agencies in preparing for licensing new reactors.”