Pushing technology’s limits to observe fundamental feature of stretched DNA
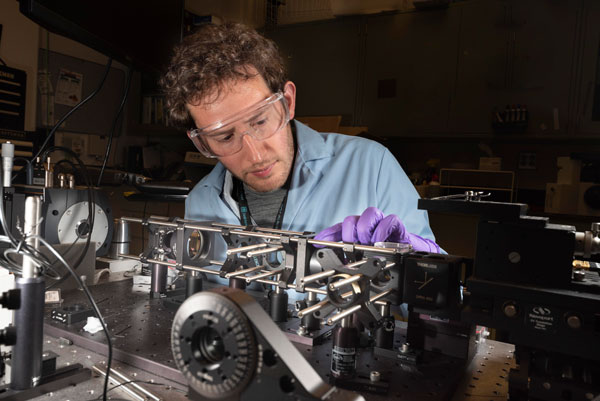
An advanced imaging technique reveals new structural details of S-DNA, ladder-like DNA that forms when the molecule experiences extreme tension. This work conducted at Sandia and Vrije University in the Netherlands provides the first experimental evidence that S-DNA contains highly tilted base pairs.
The predictable pairing and stacking of the DNA base pairs help to define the molecule’s double-helix shape. Understanding how the base pairs realign when DNA is stretched might provide insight into a range of biological processes and improve the design and performance of nanodevices built with DNA. Tilted base pairs in stretched S-DNA have been predicted previously using computer simulations, but never demonstrated conclusively in experiments until now, according to a recent article in Science Advances.
DNA is most commonly known as the molecular carrier of genetic information. However, in research labs around the world, it also has another use: construction material for nanoscale devices. To do this, scientists prepare computer-generated sequences of single-stranded DNA so that certain sections form base pairs with other sections. This forces the strand to bend and fold like origami. Researchers have used this principle to fold DNA into microscopic smiley faces, nanomachines with moving hinges and pistons and “smart” materials that spontaneously adjust to changes in the surrounding chemical environment.
“To build an airplane or a bridge, it’s important to know the structure, strength and stretchiness of every material that went into it,” said Adam Backer, a Sandia optical scientist and lead author of the study. “The same thing is true when designing nanostructures with DNA.”
While much is known about the mechanical properties of DNA’s double helix, mysteries remain about the details of its shape when the molecule is stretched in a laboratory to form the ladder-like structure of S-DNA. Standard ways of visualizing DNA structure cannot track structural changes while the molecule untwists.
Manipulating microscopic particles
To characterize the structure and stretchiness of S-DNA, Adam worked with colleagues in the Physics of Living Systems research group at LaserLaB Amsterdam at Vrije University. Adam and his co-authors, Andreas Biebricher, Graeme King, Iddo Heller, Gijs Wuite and Erwin Peterman, described their process in the journal article.
Using instrumentation developed by his colleagues, Adam first attached a microscopic bead to each end of a short piece of viral DNA. These beads served as handles to manipulate a single molecule of DNA.
Next, the researchers trapped the beaded DNA in a narrow, fluid-filled chamber using two tightly focused laser beams. Because the beads stay trapped inside the laser beams, the researchers could move the beads in the chamber by redirecting the beams. This enabled them to stretch the attached DNA to form S-DNA.
This technique for manipulating microscopic particles, called optical tweezers, also provided precise control over the amount of stretching force applied to a single DNA molecule. However, the structural changes occurring within the stretched DNA molecule were too small to be directly observed with a standard optical microscope.
Fluorescence polarization microscopy
To address this challenge, Adam helped his colleagues combine an imaging method called fluorescence polarization microscopy with the optical tweezers instrument. First, they added small, rod-like fluorescent dye molecules to the solution containing optically trapped DNA. In unstretched DNA, the dye molecules sandwich themselves between neighboring sets of base pairs and align perpendicular to the central axis of the double helix. If a stretching force causes the DNA base pairs to tilt, the dyes would also tilt.
Next, the researchers used the fluorescent signals from the dyes to determine whether the base pairs in stretched DNA tilted. The dyes emit green fluorescent light when they interact with light waves from a laser beam pointing along the same axis as the dye molecules.
The researchers changed the orientation of the light waves by rotating the polarization of a laser beam through various angles. Then they stretched the DNA and watched for green fluorescent signals to appear under the microscope. From these measurements, and computational analysis methods developed at Sandia, the researchers determined that the dyes, and thus the base pairs, aligned at a 54-degree angle relative to the DNA’s central axis.
“This experiment provides the most direct evidence to date supporting the hypothesis that S-DNA contains tilted base pairs,” Adam said. “To gain this fundamentally new understanding of DNA, it was necessary to combine a number of cutting-edge technologies and bring scientists from a range of different technical disciplines together to work toward a common goal.”
Physical principles of DNA deformation
There is widespread speculation among scientists that structures resembling S-DNA may form during the daily activities of human cells, but at present, the biological purpose of S-DNA is still unknown. S-DNA might facilitate the repair of damaged or broken DNA, helping to guard against cell death and cancer. Adam hopes this clearer understanding of the physical principles governing DNA deformation will guide further research into the role of S-DNA in cells.
When Adam joined the Labs as a Truman Fellow in November 2016, he had the opportunity to start an independent research program of his own design. He had developed a method for polarization microscopy during graduate school at Stanford University and thought the technique had potential.
“At Sandia I wanted to push this technique as far as it could go,” he said. “The fact that this work has led to results with potential relevance to fields such as biology and nanotechnology has been extraordinary.”