New MOF particles engineered to image and treat
A Sandia team has designed and synthesized nanoparticles that glow red and are stable, useful properties for tracking cancer growth and the disease’s spread.
This work is the first time the intrinsic luminescence of metal-organic framework materials, or MOFs, for long-term bio-imaging has been reported, materials chemist Dorina Sava Gallis says. Fluorescently tagging tumors, or other specific kinds of cells, is a new, powerful method to image inside a body.
MRIs, X-rays, and ultrasounds are powerful bio-imaging methods to diagnose diseases. However, these methods each have their limitations and generally aren’t used to treat diseases. For years, scientists have been searching for theranostic agents, materials that have both therapeutic and diagnostic uses.
‘Hubs’ and ‘rods’
MOFs are one group of chemicals with great potential for imaging and treating cancer and other diseases. These tinker toy-like molecules have metal “hubs” and carbon-based linker “rods.” Chemists can swap out the hubs and the linkers to make nano-sized “sponges” with many different properties. Historically, MOFs have been used for everything from capturing radioactive gases from spent nuclear fuel, to cleaning contaminated water, and even storing hydrogen gas safely.
The Sandia team’s MOF nanoparticles glow red or near infrared for at least two days in cells.
“In the field of metal-organic frameworks, we have the advantage of choosing our building blocks to make made-to-order materials.”
Near infrared light has longer wavelengths than red light. It is especially useful for imaging inside a body because it can penetrate skin, tissue, and even bone without causing damage, and produces clearer images because there’s less background autofluorescence at those wavelengths, says Dorina. Current dyes or nanoparticles that glow in the near infrared don’t last for very long or only glow weakly, making brighter, more stable materials invaluable.
MOFs are complex materials with tunable properties and astonishing surface areas; one gram of a certain kind of MOF has the same surface area as 16 basketball courts. “In the field of metal-organic frameworks,” Dorina says, “we have the advantage of choosing our building blocks to make made-to-order materials.”
For 14 years, she has been working on making the synthesis of MOFs more rational and predictable. Some metals are chemically active and others glow at certain colors. Some metals form clusters with different geometries — like tinker toy “hubs” with different numbers of holes — and sometimes the hub is a single metal ion. Some linkers are long, producing sponges with large empty spaces and high surface areas, and others are short. Some linkers are catalytically active — that is, they can speed up a chemical reaction — or can tweak the chemistry of the metal while others can adjust the color or brightness of the metal’s glow.
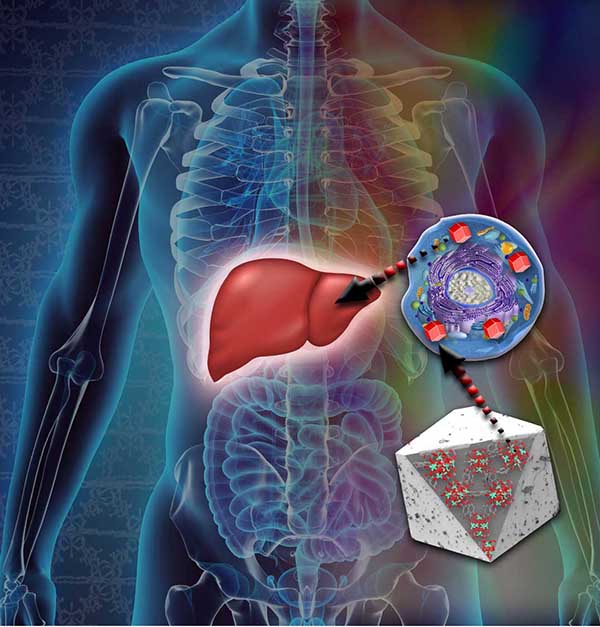
Schematic depiction of cell permeable MOF-based bioimaging agents. (Illustration by Lisa Sena-Carian)
Rational design of multifunctional MOFs for bio-imaging
To rationally design MOFs for bio-imaging, Dorina selected lanthanide metals, a class of rare earth elements. The metal europium glows red; the metals neodymium and ytterbium fluoresce in the near infrared. She also chose conditions that would cause the lanthanides to form robust clusters. Frequently, MOFs made with individual metal ions aren’t water-stable, but metal clusters often are, Dorina says. This is important for bio-imaging as cells and people are mostly water. Also, she used commonly available carbon linkers that produce large pores. Potentially, these pores could hold drugs and allow both imaging and treatment.
Dorina put together a cross-disciplinary team to confirm the MOFs had the properties she engineered. Sandia materials scientist Mark Rodriguez and Karena Chapman of Argonne National Laboratory helped with the X-ray diffraction structural studies. Sandia researchers Lauren Rohwer and Willie Luk tested the MOFs’ luminescence properties. The team successfully made a family of similar MOFs with a range of emission colors from red to near infrared allowing researchers to “tune” the MOF color depending on what it might be needed for.
Then, Dorina’s team tested to make sure the nanoparticles were stable in water and didn’t kill cultured cells. Sandia nanobiologist Kim Butler performed cytotoxicity studies to determine if the MOFs were toxic to mammalian cells. Even at high doses, the nanoparticles were similar to or less toxic than other particles being studied for bio-imaging, which is a good sign for their future, says Dorina. They were also stable in water or biology-mimicking saltwater for a least a week.
Sandia biochemist Meghan Dailey and bioanalytical chemist Jeri Timlin did live-cell imaging using a customized hyperspectral confocal fluorescence microscope. They showed the MOF particles can work for long-term bio-imaging studies in mammalian cells but might need to be further optimized, perhaps by modifying the surface of the particles, Dorina says.
“We are very excited about the success of these initial studies and are moving forward to investigate their tissue penetration depth, luminescence efficiency, and ultimately, the relevance to imaging in living organisms,” she says.
The research is part of a much larger project to develop adaptable, safe, and effective responses to biological threats and new pathogens funded by Sandia’s Laboratory Directed Research and Development program. An important part of that project is tracking the delivery of nanoparticles, which requires biologically stable glowing particles or dyes.
The results were published in ACS Applied Materials and Interfaces. The work was performed, in part, at the Center for Integrated Nanotechnologies, a DOE Office of Basic Energy Science funded user facility jointly operated with Los Alamos National Laboratory.