Sandia draws industry into quest for cheaper, cleaner electricity
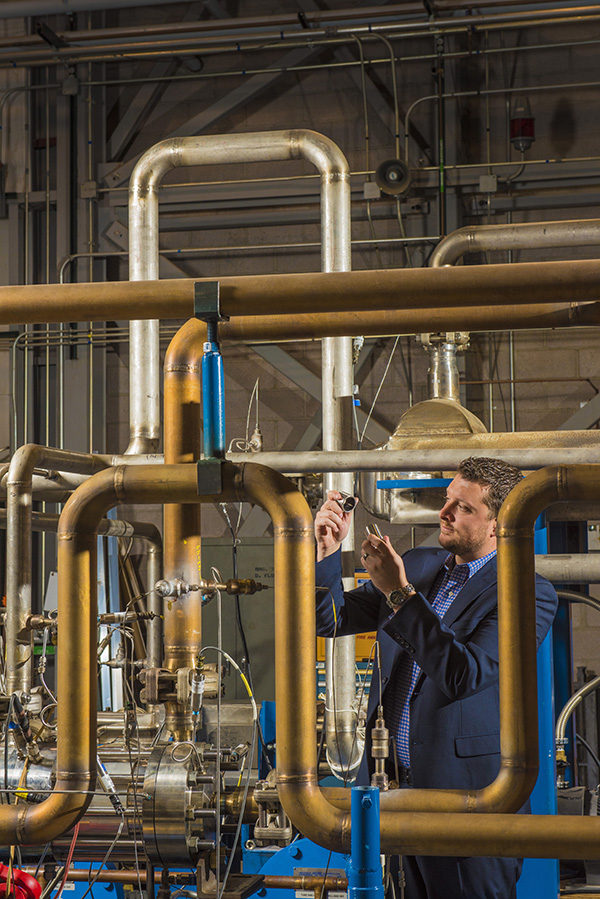
Sandia is working with three industry partners to commercialize a distributed power system that can produce cheaper, cleaner, more efficient electricity.
The Labs signed three-year Cooperative Research and Development Agreements (CRADAs) with Peregrine Turbine Technologies of Wiscasset, Maine; Xdot Engineering and Analysis of Charlottesville, Virginia; and Flowserve Corp. of Irving, Texas.
“These CRADAs give us an avenue to engage industry one-on-one for what we need and what they need, and identify a pathway to commercialization of a game-changing technology,” says Gary Rochau, manager of Advanced Nuclear Concepts Dept. 6221. “The goal is to double the efficiency of converting heat to electricity without using water and make it economical so it’s as cheap as steam.”
Sandia is developing supercritical carbon dioxide (S-CO2) recompression closed Brayton cycle technology, a power generation system that could increase thermal-to-electric conversion efficiency as much as 50 percent over conventional steam technology. Brayton cycle, named after 19th century mechanical engineer George Brayton, originally worked by heating air in a confined space and releasing it to generate shaft power or thrust, like a jet engine.
Sandia’s Brayton cycle uses S-CO2, which is chemically stable, reliable, low cost, non-toxic, non-flammable, and readily available, as the working fluid. “It’s basically a jet engine running in a closed loop with S-CO2 as the working fluid,” says Darryn Fleming (6221), principal investigator for Advanced Reactor Technology in DOE’s Supercritical Transformational Electric Power program.
Sandia has been testing various components, bearings, seals, and heat exchangers near or exceeding the critical point where carbon dioxide has the density close to a liquid but many properties of a gas. Tests showed several elements of the system were at a low technical readiness level, or TRL, a measure of technology maturity. “To get this to market, we needed to increase the TRL of numerous components and procedures, three of which are seals, bearings, and heat exchangers,” Darryn says.
Sandia posted Federal Business Opportunities ads seeking industrial partners, and Peregrine, Xdot, and Flowserve were among multiple respondents.
Challenging environment for components
Peregrine is working with Sandia on a thermally compliant heat exchanger that can handle the Brayton cycle’s rigorous thermal stresses. “We took our expertise in the design of turbo machinery hot sections and applied it to heat exchanger design to mitigate the damage normally caused by large temperature swings,” says Peregrine President David Stapp. “This design will open the door to cost-effective heat exchangers that can meet customer requirements in commercial applications.”
Xdot is developing a foil bearing that supports a turbine shaft spinning at high speed in S-CO2. “Ball bearings don’t work well in the S-CO2 environment,” says Xdot founder Erik Swanson. “We have a foil bearing that can be directly lubricated by S-CO2 with better performance at lower cost — a better mousetrap.”
Flowserve’s high-intensity seal for power turbines is designed to hold tight with S-CO2 going into the turbine at 700 degrees C and 4,400 pounds per square inch, or psi. “That is super high temperature, super high pressure,” Darryn says. “Seals can be leaky. We need a seal that won’t bleed out and send CO2 into the atmosphere.”
Lionel Young, director of advanced technology for Flowserve, says his company started from scratch on a seal to perform in S-CO2. “Although the high pressure and speeds for this application are not new for Flowserve, such a seal hadn’t been designed for these extreme temperatures,” he says. “The critical part has been the design of the secondary dynamic gasket. The seal for the most part relies on conventional technology but the dynamic gasket, which must be able to slide and seal, has to be able to work at these extreme pressures and temperatures without leakage.”
Ability to test at high temperature and pressure
Sandia offers its partners a 1-megawatt thermal S-CO2 recompression closed Brayton cycle test loop that can run dynamic tests along with dedicated test rigs for bearings and seals. “We help them test their technologies to prove they work with supercritical CO2. They have the expertise but not the testing ability. Going to temperatures and pressures that far exceed typical metals is not trivial,” Darryn says. “High temperature and pressure testing with supercritical CO2 is complex and testing is expensive and high risk. We’re set up to do multiple large-scale demonstrations that use high temperature and high pressure. It helps get their products out to market faster and with less risk.”
All three companies have made strides and developed prototypes with Sandia’s technical input and testing made possible by the CRADAs, agreements between a government agency and a private company or university to work together on research and development.
“It’s been great to work with a national lab,” Swanson says. “They have incredible resources. We’re sharing our technology and as the program goes on we look forward to the test data for this challenging application.”
Young says Flowserve has limited ability to test at the temperature requirements of S-CO2 Brayton cycle. “While Flowserve will test a prototype at full pressure and speed, but at a lower temperature, we will depend on Sandia to test to full temperature,” he says. “The collaboration between our team and Darryn and his team has been excellent. They are open and helpful and we believe the result will be to help advance seal technology.”
Stapp says being teamed with Sandia’s experts has been invaluable. “I couldn’t be happier,” he says.
Aiming for a 10-megawatt demonstration
The goal of Sandia and DOE is to have a 10-megawatt commercially demonstrated S-CO2 Brayton cycle at the Labs by 2020. “It’s now time to take this technology and build test rigs,” Darryn says. “A 10 megawatt electric demonstration needs to be made. That’s where the commercial entities get excited. They’re not interested in small laboratory units. We need a commercially scalable system.”
The Brayton technology can use solar, biofuels, and natural gas to produce electricity for the distributed energy market, Gary says. Distributed electrical generation is the production of power at or near point of use versus at a large-scale, central generation location requiring long-distance transmission and local distribution. The cost of transmission and distribution typically accounts for half the cost of delivered electricity.
Gary says Sandia is working through the three CRADAs with manufacturers who will bring Brayton technology to market, US produced, manufactured and exportable. “We’re helping them and they’re helping us,” he says. “If they see the market and we have the science, we’re getting the engineering done so they can hit the market, and that cranks ?everything up. We don’t want a novelty that nobody can use.”
What’s in a power cycle?

Most electricity produced around the world uses a general closed-loop cycle that pressurizes a fluid, heats it so it has a lot of expansion capability, then blows it through a turbine that operates a generator. The low-pressure fluid exits the turbine, is condensed by removing heat, pressurized again, and reused in the cycle.
The most common cycle, Rankine, boils pressurized water to create steam, which is then expanded through the turbine. The cycle efficiency is about 33 percent — that is, 33 percent of the thermal energy delivered to the fluid is converted into electricity.
Sandia’s Brayton cycle uses supercritical carbon dioxide (S-CO2) as the working fluid, instead of steam, increasing conversion efficiency up to 50 percent, says Darryn Fleming (6221). S-CO2 is a fluid state of carbon dioxide where it is held above its critical pressure and critical temperature, causing the gas to go beyond liquid or gas into a phase where it acts as both simultaneously.
“One percent of efficiency in a power plant in today’s market translates into millions and millions of dollars because less fuel is burned to make the same amount of electricity,” Darryn says. “A one-percent improvement in efficiency also reduces greenhouse gases by about 2.9 percent. Increasing efficiency to 50 percent reduces emissions by 34 percent. Consumer costs will decline as efficiency improves and fewer natural resources are consumed.”
The reason for higher efficiency is that a sensible temperature difference between the hot turbine discharge and the cold compressor discharge drives heat transfer within the cycle, which provides the vast majority of the heat addition to the high-pressure fluid. The heat rejected while condensing steam at constant temperature in a Rankine cycle is avoided.
While the process of internal heat recuperation applies to any gaseous working fluid, S-CO2 remains relatively incompressible and dense at normal atmospheric temperatures so the low-temperature condition in the cycle that minimizes the work of compression is easily and cheaply achieved, Darryn says.
Brayton cycle turbines would typically be used for bulk thermal and nuclear generation of electricity, including next-generation power reactors. The goal is to replace steam-driven Rankine cycle turbines, which have lower efficiency, are corrosive at high temperature, and occupy 30 times as much space because of the need for very large turbines and condensers to dispose of excess steam.
There is a lot of industrial and scientific interest in S-CO2 systems for power generation because they can use any heat source including solar, geothermal, fossil fuel, biofuel, and nuclear. “An ideal place to put a solar plant is in the desert, but there’s no water,” Darryn says. “Our Brayton cycle is fuel agnostic. You can use nuclear, fossil, renewables. You can use any type of heat source without the use of water because of dry heat rejection.”