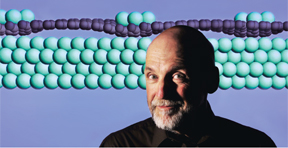
Graphene flakes are notoriously difficult to work with. Still, they are stronger than diamond, better heat-shedders and conductors than silicon, and thought to have great potential in the worlds of microelectronics and sensors.
In 2005, a German team discovered a new wrinkle in the battle to harness them. A graphene flake lying atop an iridium crystal unexpectedly caused new iridium atoms, deposited on top of the flake, to arrange themselves into equally sized, equally spaced clusters. Not only that — the cluster arrays remained stable even as the temperature was raised into the 400 to 500 kelvin range.
Imagining a whole new set of possible applications, people wanted to know why.
It was hard to understand how a graphene sheet — a featureless, flat sheet of carbon atoms — lying on an equally featureless iridium surface, somehow converted itself into a kind of muffin tin that drew newly arrived iridium atoms into equally spaced, equally sized clusters (“muffins”).
“At the outset,” writes Sandia researcher Peter Feibelman (1130), “this seemed quite a mystery.”
Sherlock Holmes himself, looking for clues to why the iridium quantum dots so mysteriously attached, would have found little to go on.
The iridium support layer was flat as could be. The same was true of the graphene layer that formed on top of it, which sported neither hooks nor ports for nanoparticle docking.
Graphite itself — merely a group of sheets of graphene — is so slippery it can be used as a lubricant. Why would nanodots attach to the completed graphene layer instead of just sliding away?
Even granted an attachment mechanism, why would newly introduced iridium atoms form a moiré — a regular, ordered array — atop the graphene instead of a planar second surface — a sandwich where the iridium was the bread and graphene the meat?
The explanation for the template effect would be almost impossible to see by direct examination.
But Peter’s computational simulations, detailed in a paper published electronically last week by Physical Review B, produced a plausible explanation.
His work demonstrates that in regions where half the graphene flake’s carbon atoms sit directly above iridium atoms of the underlying crystal, iridium atoms added on top of the graphene flake make it buckle. These regions do not occur randomly, and in fact form the regular array needed to explain the nanodot moiré.
The buckling weakens tight links between the graphene’s neighboring carbon atoms, freeing them to attach to the added iridium atoms. Furthermore, buckling not only allows the carbon atoms that buckle upward to capture deposited iridium atoms, but also causes the carbon atoms that buckle down to attach firmly to the metal below, explaining the remarkable thermal stability of the nanodot arrays.
This orderly nanoscopic arrangement appeals to scientists trying to understand aspects of catalysis, Peter says. The atoms that make up tiny nanodots are expected to be in direct contact with inserted materials, important for speeding up desirable chemical reactions. The regular arrangement of the nanodots makes the science relatively simple, because every catalyst particle is the same and sits in the same environment.
“The rigorous periodicity of the nanodot arrays is a huge advantage compared to amorphous or ‘glassy’ arrangements where everything has to be described statistically,” says Peter.