Researchers explore making ammonia with little more than sun, air and hydrogen
Sandia scientists are working to make feeding the world much less carbon intensive by developing a new chemical-producing process.
About half the world’s food production relies on nitrogen fertilizer primarily composed of ammonia synthesized via the Haber-Bosch process. This process uses fossil fuels made of hydrocarbons to drive the reactions that convert hydrogen and nitrogen into ammonia for fertilizer, medicine, plastics and other synthetic products. Haber-Bosch ammonia production accounts for almost 1.5% of global carbon dioxide emissions, the main driver of global climate change.
“We’ve been making ammonia the same way for almost 100 years,” said Andrea Ambrosini, an inorganic chemist in Sandia’s concentrating solar technologies department. “Conventional ammonia production via the Haber-Bosch cycle requires high pressures and temperatures to efficiently react nitrogen and hydrogen. This is a very energy- and carbon-intensive process.”
Andrea leads a team of Sandia researchers collaborating with partners at Arizona State University and the Georgia Institute of Technology. Together, they are exploring ways to decarbonize ammonia production by using concentrated solar thermal power. Their work is funded by DOE’s Solar Energy Technologies Office.
“We’re leaders in concentrating solar power and have the only concentrating solar power tower test facility in the United States,” Ambrosini said, referring to Sandia’s National Solar Thermal Test Facility. “Currently, CSP is mainly used to produce heat for electricity generation, but a natural progression is to explore using CSP-generated heat in other ways, like splitting water to produce hydrogen or producing ammonia.”
The team developed — and submitted a patent for — the Solar Thermal Ammonia Production, or STAP, process, which uses advanced solar thermochemical looping technology to purify nitrogen from the air and synthesize ammonia under relatively low pressures. “Basically, we’re trying to create a synthesis method for ammonia that uses sunlight rather than hydrocarbons,” Ambrosini said.
Shining light on novel materials
The first step of this new potentially carbon-free, renewable process addresses emissions from current methods for producing the pure nitrogen needed to synthesize ammonia. In addition to using concentrated solar energy, rather than fossil fuels to generate heat, the team turned to materials science in search of a greener alternative to generate nitrogen, which is currently sourced from greenhouse gas-emitting hydrocarbons.
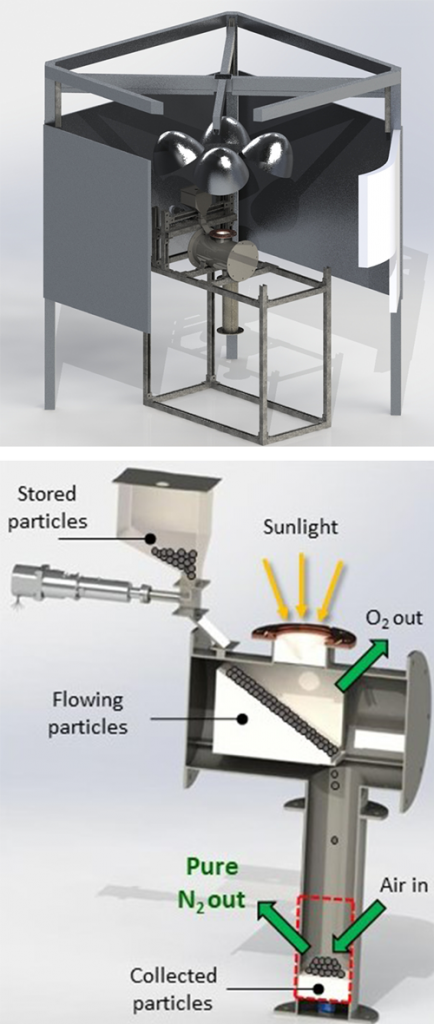
The research team developed a metal oxide material that thermally reduces, releasing some of its oxygen when heated. “This primes the material to pull oxygen out of the air flowing over it, producing almost pure nitrogen gas,” said Evan Bush, who started as a postdoctoral researcher on this project and is now a full-time Sandia staff member.
A solar simulator at Sandia will be used to test the metal oxide reduction reaction when heated with concentrated light. “You flip a switch to turn on lamps that concentrate light into a very small space,” Bush said. “This means you don’t have to worry about solar intermittencies or time of day. It’s a good test-bed system to prove concepts before moving to a bigger scale.”
Like the first step, the second step of the team’s STAP process relies on novel materials. Metal nitrides are needed to react in a low-pressure looping process that yields a greener, renewable pathway for producing ammonia.
“This step is an important area for novel materials research because these nitride materials are much less known,” Bush said. The team tests small — less than one gram — quantities of candidate nitrides under specific conditions to determine their ammonia-production potential. When a promising nitride is identified, larger samples of one to two grams are synthesized and tested in a benchtop prototype reactor.
In one such reactor, constructed and used at Arizona State University, the metal nitride is exposed to hydrogen gas, which reacts with nitrogen in the material to produce ammonia at a pressure level about one order of magnitude lower than the level required in the Haber-Bosch process.
To replace the lost nitrogen, the metal nitride is replenished with the high-purity nitrogen generated during the first step of the process. This uptake of nitrogen closes the cycle, returning the material to its original state. Since the particles in both the nitrogen and ammonia-production steps are not consumed as part of the overall process, both the metal oxide and metal nitride can be recycled to repeat the loop.
The result? Ammonia made from little more than sun, air and hydrogen.
If that last input — hydrogen — can also be renewably sourced, this would decrease ammonia’s carbon footprint even more. While not part of this project, efforts at Sandia and elsewhere are underway to produce green hydrogen by splitting water with solar energy.
From lab to field
Although Ambrosini’s team has demonstrated proof of concept, they have not yet physically coupled the first and second steps of the STAP process. Coupling these two steps, as well as scaling up materials synthesis and reactor designs from the lab to a large-scale demonstration, is the next challenge in developing this low-carbon technology.
“Eventually, you would want to develop reactors that sit on top of a solar tower and have a heliostat field, or field of mirrors, concentrating the sunlight to run the metal-oxide priming process,” Bush said, adding that the total amount of ammonia produced would depend on the size of the reactors.
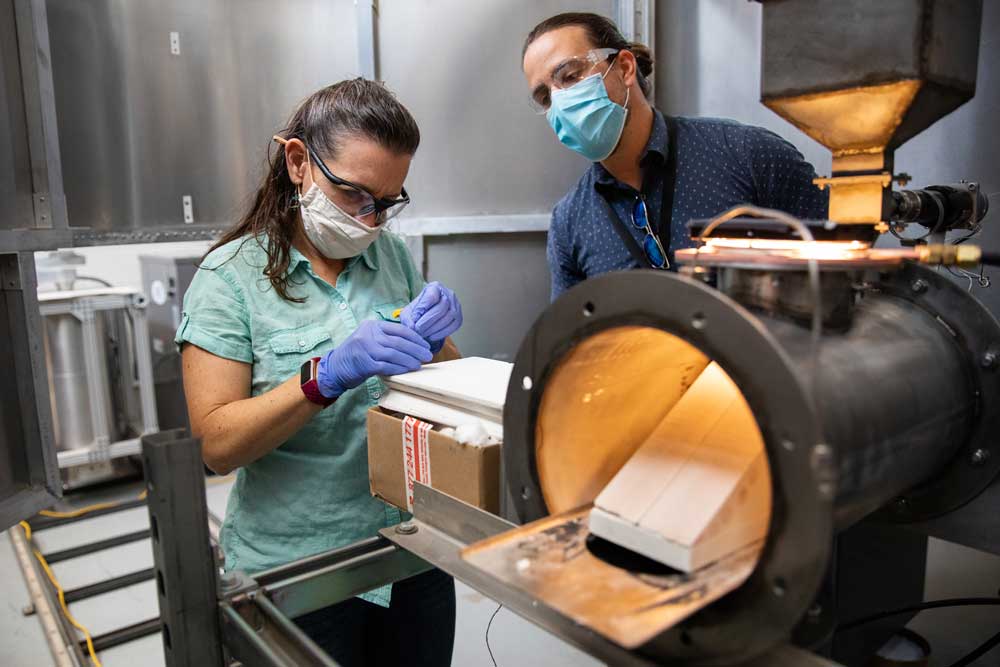
In addition to providing research expertise, Sandia brings a unique capability to the overall research team: access to the National Solar Thermal Test Facility, which includes a solar furnace and the full-scale concentrating solar tower, for testing the STAP process at a greater scale.
To take advantage of economies of scale, Haber-Bosch ammonia facilities are typically quite large. Large-scale production will remain important, explained Andrea, but ideally, the STAP process could also be deployed more locally. Producing ammonia where it is needed —for example, by locating a smaller-scale plant closer to agricultural land — would further reduce carbon emissions by decreasing the need to transport ammonia over long distances.
Ambrosini said more research is needed to understand the basic mechanisms by which the novel materials make ammonia. “Figuring out exactly how these materials work would be very interesting. There may be even better material out there that we haven’t studied yet.”
Bush said, “There’s definitely more work we can do in terms of figuring out the optimal material to run the ammonia-formation step. We’re seeking a balance between what produces a lot of ammonia and what is actually cost-competitive.”
As part of this project, their collaborators at Arizona State University are conducting a techno-economic analysis to better understand the overall benefits and costs of this new process.
The lessons learned from producing ammonia with concentrated solar energy could also be applied to other chemical-making processes, such as producing synthetic fuels or hydrogen, said Bush. “The things we learn will expedite the development of all sorts of different processes, including materials generation and solar electricity production from concentrating solar power.”
Share this story:
August 31, 2022